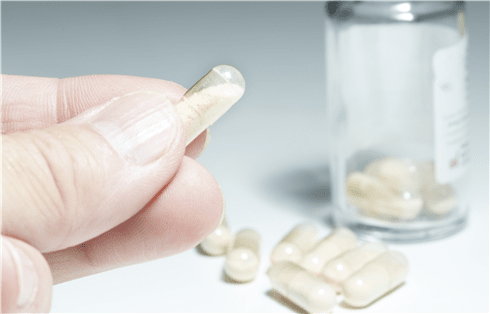
Existing research shows that nucleic acids can be used for the treatment of neurological diseases, cardiovascular diseases, and cancer, as well as for the prevention of chronic and infectious diseases. They can also be used to correct genetic material through gene editing to cure rare diseases. Therefore, nucleic acid drugs can expand the targets and space for disease treatment, which is of great significance for human medical health.
Nucleic Acid Drugs and Their Mechanisms
Nucleic acid drugs are usually composed of natural or modified deoxyribonucleotides or ribonucleotides, including DNA, RNA, antisense oligonucleotides (ASOs), and nucleic acid aptamers. RNA includes small interfering RNA (siRNA), microRNA (miRNA), short hairpin RNA (shRNA), messenger RNA (mRNA), self-amplifying RNA (saRNA), circular RNA (circRNA), etc.
DNA is a double-stranded polymer of deoxyribonucleotides. Circular plasmid DNA can guide the translation and synthesis of target proteins through mRNA transcription in the cell nucleus. The molecular weight of DNA depends on the size of the expressed protein. The target site for DNA drug delivery is the nucleus, so the requirements for delivery systems and technologies are relatively rigorous, which is the most challenging part in the development of nucleic acid drugs. The advantage of DNA as a nucleic acid drug is its good stability, and once it enters the cell nucleus, its translation and regulation of target proteins are more persistent than mRNA.
ASOs usually consist of 15-25 nucleotides. Single-stranded ASOs need to be chemically modified to improve their stability, reduce immunogenicity, and improve in vivo behavior. The modified sites are usually on the sugar cycle and phosphoric acid skeleton. ASOs interact with the target mRNA through Watson-Crick base pairing principles. The interaction can occur in the cytoplasm or nucleus. The mechanisms by which ASOs achieve target protein downregulation are divided into the following two types:
- Through RNA-DNA hybrid design, it binds to the target mRNA and recruits ribonuclease H (RNAse H) to achieve specific degradation of the target mRNA.
- By specifically blocking the binding of splicing proteins and pre-mRNA, it can block the processing from pre-mRNA to mature mRNA, thereby regulating the target protein.
Nucleic acid aptamers are single-stranded nucleic acids with a length of 50-120 nucleotides. Nucleic acid aptamers include DNA aptamers and RNA aptamers. Although composed of DNA or RNA like other nucleic acids, the target of aptamers is neither on pre-mRNA nor on mRNA, but directly on the target protein. They block the corresponding function of the protein by spatial hindrance.
siRNA is a typical representative of the RNA interference mechanism, which can achieve efficient silencing of target proteins. siRNA is a double-stranded RNA composed of a sense chain and an antisense chain. The target site for siRNA is in the cytoplasm. siRNA in the cytoplasm can composite with RNA-induced silencing complex (RISC). After the complex, siRNA undergoes double-stranded denaturation, and the sense chain leaves the RISC complex, while the antisense chain continues to composite with the RISC complex. The antisense chain contains a base sequence complementary to the target mRNA, so it can guide the RISC complex to bind to the target mRNA. The RISC complex contains nucleic acid degradation enzyme Argonaute. When the antisense chain of siRNA binds to mRNA, the Argonaute enzyme in RISC will degrade mRNA, thereby reducing the translation of mRNA into the target protein.
mRNA can up-regulate the expression of target proteins in the body. mRNA works in the cytoplasm and binds to ribosomes to translate target proteins. The target protein translated by mRNA can be an intracellular protein or can be secreted by cells, thereby being taken up by other cells (such as antigen-presenting cells) or specifically interacting with target points on the cell surface. The stability of mRNA in vivo and in vitro is relatively poor, and it usually has a shorter persistence in action in cells than DNA.
Properties and Delivery Barriers of RNA Nucleic Acid Drugs
The physical and chemical properties of nucleic acid drugs are different from those of traditional small-molecule drugs mainly in terms of molecular weight and hydrophobicity. Nucleic acids are usually composed of multiple nucleotides linked together by phosphodiester bonds, with nucleotide units ranging from 20 to 10,000. Among them, siRNA consists of about 20-30 nucleotides and belongs to the category of small nucleic acids, but its molecular weight is also as high as about 13 kD. Small nucleic acids can generally be obtained through chemical synthesis.
The number of nucleotides in mRNA is related to the size of the protein it encodes, ranging from 500 to 5000, and the molecular weight can reach 1000 kD. Under the same coding protein, saRNA (self-amplifying RNA) is usually larger than mRNA, up to 9,500 base pairs, mainly because saRNA contains some self-amplifying elements.
These self-amplifying elements can significantly reduce the use of nucleic acid doses. The target sites for mRNA and saRNA delivery are both in the cytoplasm, and they can be synthesized through in vitro transcription. Since the synthesis process does not require cells, the synthesis and preparation process is relatively simple. Moreover, compared with antibody and protein drugs, it can avoid cell-derived impurities, and the purification process is relatively simple. Due to the large molecular weight of nucleic acid drugs, there are many sites that molecules can attack and destroy, so their stability is poor, and they are easily degraded in vivo. Also, they are not easily accessible to cells and subcellular organelles to play a role. In addition, the phosphodiester backbone of nucleic acid drugs makes them highly negatively charged and hydrophilic, making it difficult for them to cross the cell membrane, resulting in extremely low delivery efficiency in vivo. Furthermore, RNA itself can be recognized by the body’s pathogen-associated molecular patterns (PAMPs), which can activate the body’s innate immune system, leading to the rapid clearance of nucleic acids in the body and reducing their utilization rate.
The in vivo delivery of nucleic acid drugs faces many barriers, including extracellular barriers and intracellular barriers. Extracellular barriers include plasma protein complexes in blood, mononuclear phagocyte system, plasma nucleic acid degradation, liver metabolism, kidney excretion, etc. Intracellular barriers refer to nucleic acid transmembrane transport. After entering the cell, it is wrapped by the endosome, and as the endosome matures, the pH of the endosome gradually decreases and becomes acidic. At the same time, various enzymes in the endosome-lysosome system are activated under acidic conditions, ultimately leading to the degradation and destruction of nucleic acids. Studies by the Max Planck Institute (MPI) and Alnylam on siRNA-LNP have found that the vast majority of siRNAs remain in the endosome-lysosome system, and only about 1%-2% of siRNAs can escape from the lysosome and enter the cytoplasm to play a role. This quantitative study clarifies the importance of lysosomal escape efficiency in the pharmacological properties of nucleic acids. There are many strategies to increase the lysosomal escape efficiency of nucleic acids, such as peptides, cationic lipids, cationic polymers, polyethyleneimine, polyamide-amines, cationic nanosuspensions, etc. However, the choice of lysosomal escape strategy must consider safety in clinical applications and avoid adverse reactions that may be caused by delivery agents, carrier materials, and their metabolites. Therefore, how to balance delivery efficiency and safety has always been a focus and difficulty in the field of nucleic acid delivery.
Delivery Systems for RNA Nucleic Acid Drugs
Clinical delivery vehicles for RNA nucleic acid drugs can be mainly divided into three categories: lipid nanoparticles (LNP), polymer-based nanoparticles, and lipid-polymer hybrid nanoparticles. However, currently verified delivery vehicles in the real world are mainly based on ionizable lipids for LNP. The biggest difference between this carrier and traditional cationic lipid nanoparticles is that LNP uses ionizable lipid molecules as its core lipid, which makes LNP electrically neutral under physiological conditions, but can present a positive charge through protonation in lower pH environments, such as acidic buffer solutions and the endosomal-lysosomal system. The characteristics of this ionizable lipid can help to seal RNA molecules in vitro and achieve effective lysosomal escape in vivo. Traditional cationic lipid nanoparticles, polymer-based nanoparticles, and lipid-polymer hybrid nanoparticles typically use cationic lipids or cationic polymers, which have permanent positive charges. Although these materials can also achieve RNA encapsulation and delivery, the cationic groups they contain will interact electrostatically with various negatively charged proteins, lipids, and cells inside the body during the delivery process, causing tissue and cell toxicity and activating the reticuloendothelial system. Therefore, the key advantage of ionizable lipids over traditional cationic materials is that the former largely solves the problem of balancing delivery efficiency and safety in vivo.
RNA Nucleic Acid Drugs on the Market
Currently, multiple RNA nucleic acid drugs have been approved by the US FDA and the European Medicines Agency (EMA) and are on the market. These drugs can be used not only for targeting the liver but also for the eyes, brain, muscles, and lymphatic systems. RNA nucleic acid drugs currently have a wide range of therapeutic applications, such as antiviral retinitis, familial hypercholesterolemia, spinal muscular atrophy, Duchenne muscular dystrophy, multiple neurological diseases caused by amyloidosis, hyperlipidemia, acute hepatic porphyria, hyperoxaluria, and COVID-19 vaccines.
Formulation | Onpattro | Pfizer/BioNTech COVID-19 Vaccine | Moderna COVID-19 Vaccine |
Nucleic acid | siRNA | mRNA | mRNA |
Dose | 10 mg in 5mL | 30 μg in 0.3 mL | 100 μg in 0.5 mL |
Composition | MC3/DSPC/Chol/DMG-PEG2k | ALC-0315/DSPC/Chol/ALC-0159 | SM102/DSPC/Chol/DMG-PEG2k |
Molar ratio | 50/10/38.5/1.5 | 46.3/9.4/42.7/1.5 | 50/10/38.5/1.5 |
N/P ratio | 5 | 6 | 5 |
DSPC: 1,2-Distearoyl-sn-glycero-3-phosphocholine; Chol: Cholesterol; DMG-PEG2K: 1,2-Dimyristoyl-rac-glycero-3-methoxypolyethylene glycol-2000
Conclusion and Outlook
RNA nucleic acid drugs are a new generation of biotechnology drugs, and their platformization and application in disease prevention and treatment require the joint efforts of scientists in various fields. It is believed that with further research on issues such as in vivo and in vitro stability, in vivo fate and safety, and tissue targeting, RNA nucleic acid drugs will demonstrate strong vitality in the prevention and treatment of major emerging infectious diseases and in the resolution of major clinical unmet needs, genetic editing, and gene therapy.